Quantum computing is set to revolutionize the technological landscape, particularly in hardware design. Unlike classical computers, which rely on binary bits (0s and 1s) for processing information, quantum computers use qubits, allowing for parallel computation and unprecedented processing power. This shift is expected to bring a paradigm change in how hardware is structured, optimized, and deployed across industries.
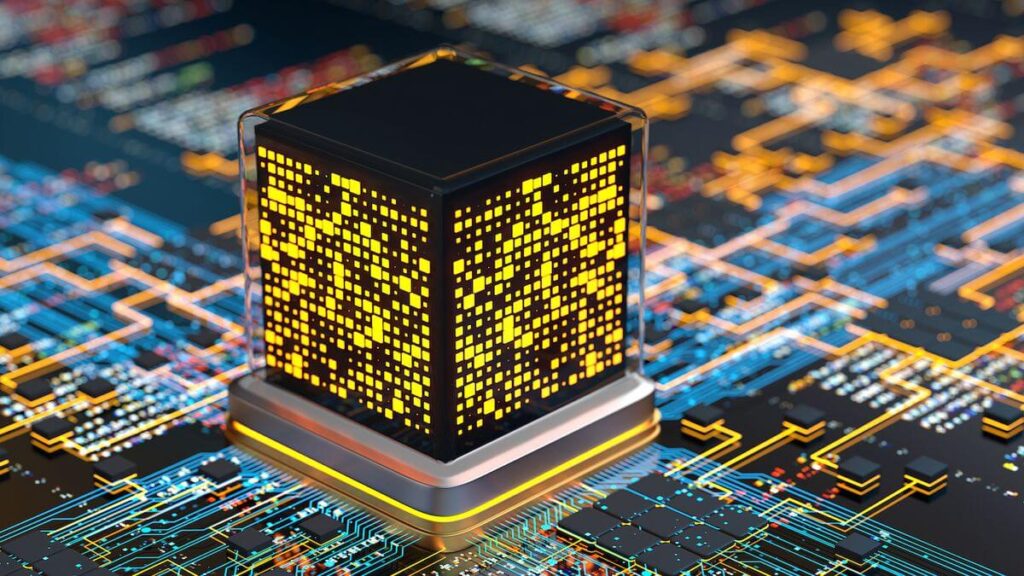
As technology companies push the limits of classical computing, the demand for next-generation hardware capable of handling quantum algorithms is increasing. Major players like IBM, Google, and Intel are investing heavily in quantum research (IBM Quantum), fueling innovations that will redefine traditional hardware architecture. The integration of quantum computing into mainstream technology will not only enhance computing capabilities but also introduce new materials, chip designs, and infrastructure models.
The Shift from Classical to Quantum Hardware
Classical computing has long depended on silicon-based processors and transistor scaling as defined by Moore’s Law. However, as transistors shrink to atomic scales, physical limitations hinder further performance improvements. This has led researchers to explore alternative computing models, with quantum computing emerging as a viable solution.
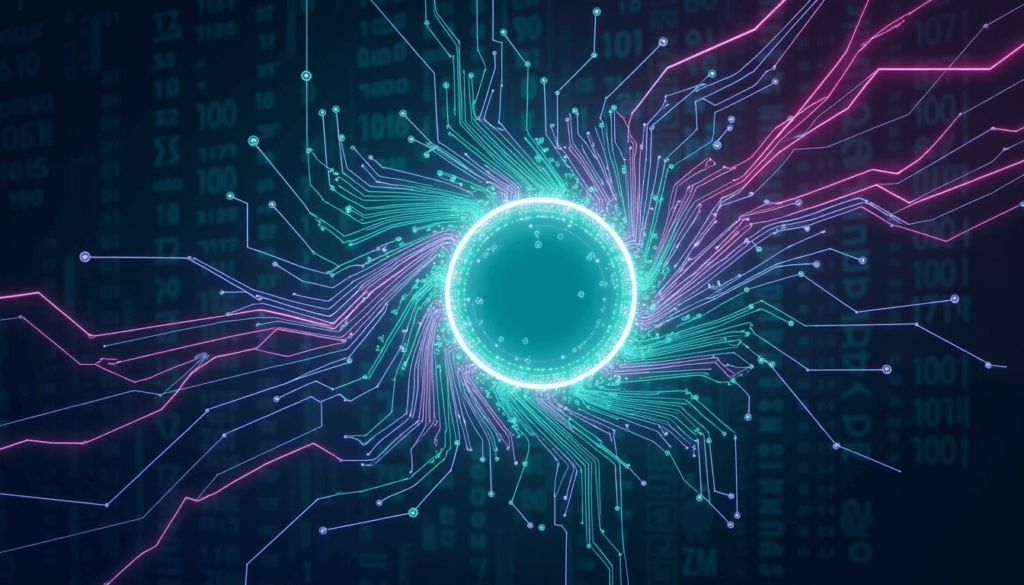
Quantum processors operate using quantum superposition and entanglement, allowing them to process vast amounts of data simultaneously. Unlike traditional CPUs and GPUs, quantum hardware relies on superconducting circuits, trapped ions, and photonic processors. These new architectures demand specialized cooling systems, novel error correction methods, and quantum-specific programming languages. The transition from classical to quantum hardware will drive fundamental changes in the semiconductor industry, influencing processor manufacturing, storage systems, and computational methodologies (Intel Quantum Computing).
Impact on Chip Design and Semiconductor Industry
The introduction of quantum computing will drastically alter chip design. Traditional processors rely on increasing transistor counts to boost performance. In contrast, quantum processors require entirely different architectures that leverage quantum gates and circuits. Companies such as Intel are developing hybrid chips that integrate classical and quantum computing elements to enable a smoother transition to quantum-era processing.
Moreover, the semiconductor industry will see an increased demand for materials like superconductors, topological insulators, and exotic elements that enable stable qubit operation. As quantum technology advances, chip manufacturers must rethink fabrication techniques and explore new materials that support quantum coherence and minimize decoherence effects (Semiconductor Industry Association).
The Role of Quantum Computing in AI and Big Data
Quantum computing’s ability to process massive datasets at unprecedented speeds makes it a game-changer for artificial intelligence (AI) and big data analytics. Traditional AI models often face computational bottlenecks, particularly in deep learning and neural network training. Quantum hardware can overcome these limitations by enabling faster optimization, more efficient pattern recognition, and improved machine learning algorithms.
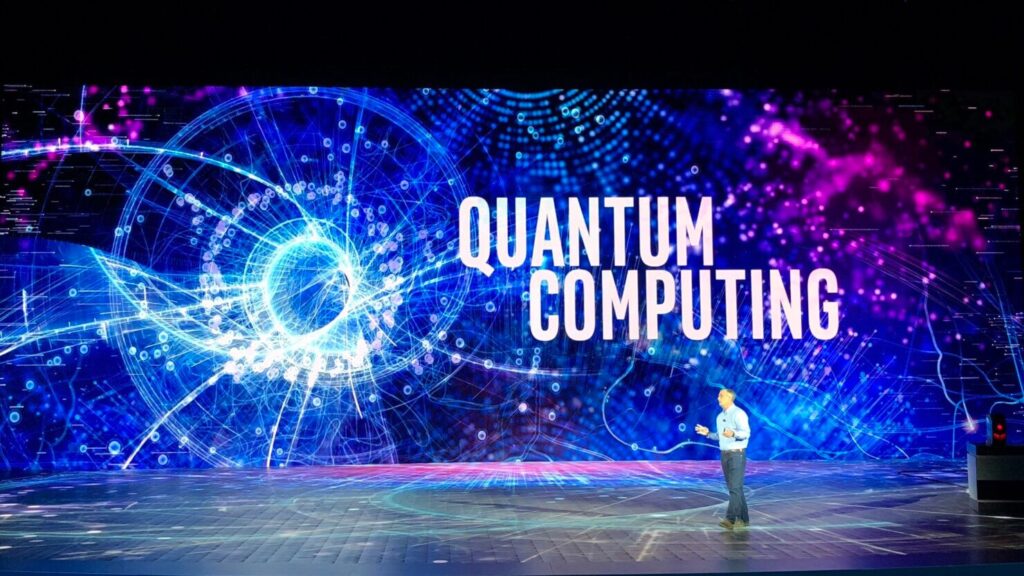
For instance, companies like Google AI and Microsoft are developing quantum-enhanced machine learning frameworks that leverage quantum principles to accelerate training times and improve accuracy. The fusion of AI and quantum computing will lead to more powerful autonomous systems, enhanced cybersecurity, and breakthroughs in fields like drug discovery and climate modeling (Google Quantum AI).
Challenges in Quantum Hardware Development
Despite its potential, quantum computing faces several hardware-related challenges that must be addressed before widespread adoption. One of the primary hurdles is qubit stability. Qubits are highly susceptible to environmental disturbances, which lead to decoherence and computational errors. Researchers are exploring error-correcting codes, quantum error mitigation techniques, and hardware improvements to extend qubit lifespan and reliability.
Additionally, quantum computers require ultra-low temperatures to maintain qubit coherence, necessitating advanced cryogenic cooling systems. The development of room-temperature quantum hardware remains a critical goal for making quantum technology more accessible and practical for everyday applications.
The Future of Quantum-Optimized Hardware
As quantum computing advances, its integration into mainstream hardware will follow a phased approach. In the near term, hybrid computing systems combining classical and quantum processors will become the norm. Cloud-based quantum computing services from IBM Quantum and Amazon Braket already allow developers to experiment with quantum algorithms without requiring dedicated quantum hardware.
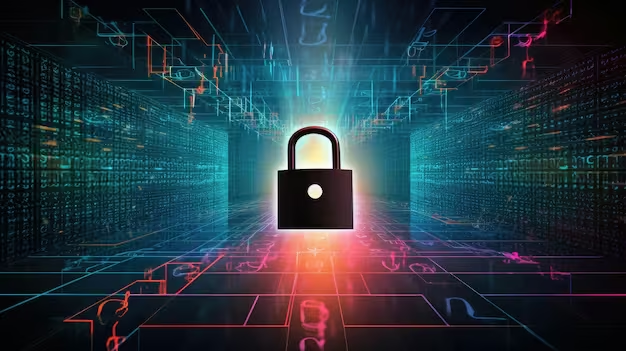
Looking further ahead, breakthroughs in fault-tolerant quantum computing, quantum networking, and scalable qubit architectures will pave the way for fully functional quantum computers capable of outperforming classical supercomputers. The impact on industries such as finance, cryptography, pharmaceuticals, and logistics will be profound, unlocking solutions to previously intractable problems.
Conclusion
Quantum computing represents the next frontier in technological innovation, with profound implications for hardware design and computing power. As researchers and companies continue to refine quantum processors, the way we approach hardware engineering will undergo a fundamental transformation. The road ahead presents challenges, but the potential for exponential growth in computational capabilities makes the pursuit of quantum hardware an essential endeavor for the future of technology.